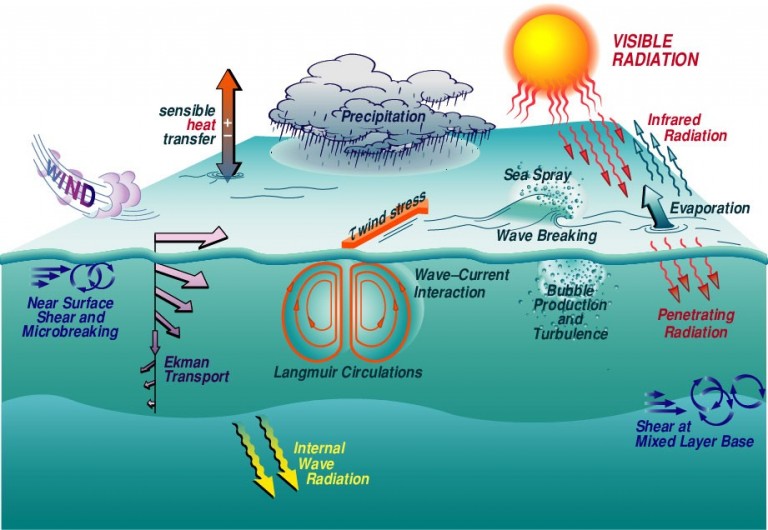
Two focal pieces of equipment are being deployed from Falkor during the Mixing Up the Tropical Pacific cruise. One is the Vertical Microstructure Profiler (VMP), which measures microconductivity (tells you how salty the water is), temperature, and shear. The other is the Acoustic Doppler Current Profiler (ADCP), which also generates data pertaining to shear. Shear is an interesting component of oceanography; so let’s learn what shear is and why it is important, so we can better understand the data from the instruments.
Shear
When we talk about the importance of shear in ocean mixing, we are not including the surface “mixed layer,” which may become blended by other atmospheric processes such as wind (shown in the figure above). Shear only pertains to the layers at and below the base of the “mixed layer”, and is the driving component of mixing here. In typical subsurface ocean conditions, layers closer to the surface are lighter than layers underneath, and mixing is accompanied by an increase in the overall potential energy of the flow due to the energy required to overcome this density difference.
Changes in center of gravity
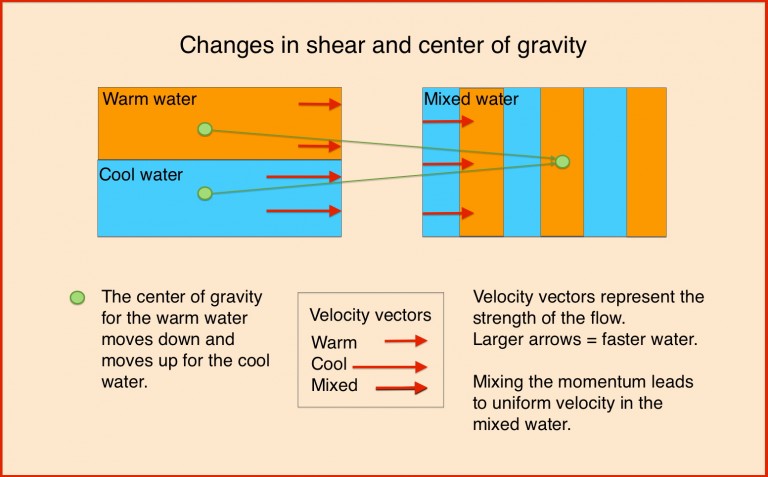
Unmixed layers have different centers of gravity—the depth in the middle of the layer. Mathematically, two layers of fluid can be considered mixed if their centers of gravity coincide. With some assistance from Dr. Andrei Natarov, I produced a figure (shown on the left) to represent these changes. The amount of energy required to raise the heavier water is more than the potential energy released by lighter water moving downward. You can think of it like this: it takes more energy to lift a car than to drop a bicycle, no matter how relieved you feel to release the bike. Dropping the bike represents an energy gain, but you still need more than that gained energy to lift the car. A lot more. Similarly, mixing often requires more energy for the colder, denser layer to move its center of gravity upward.
Converting energy
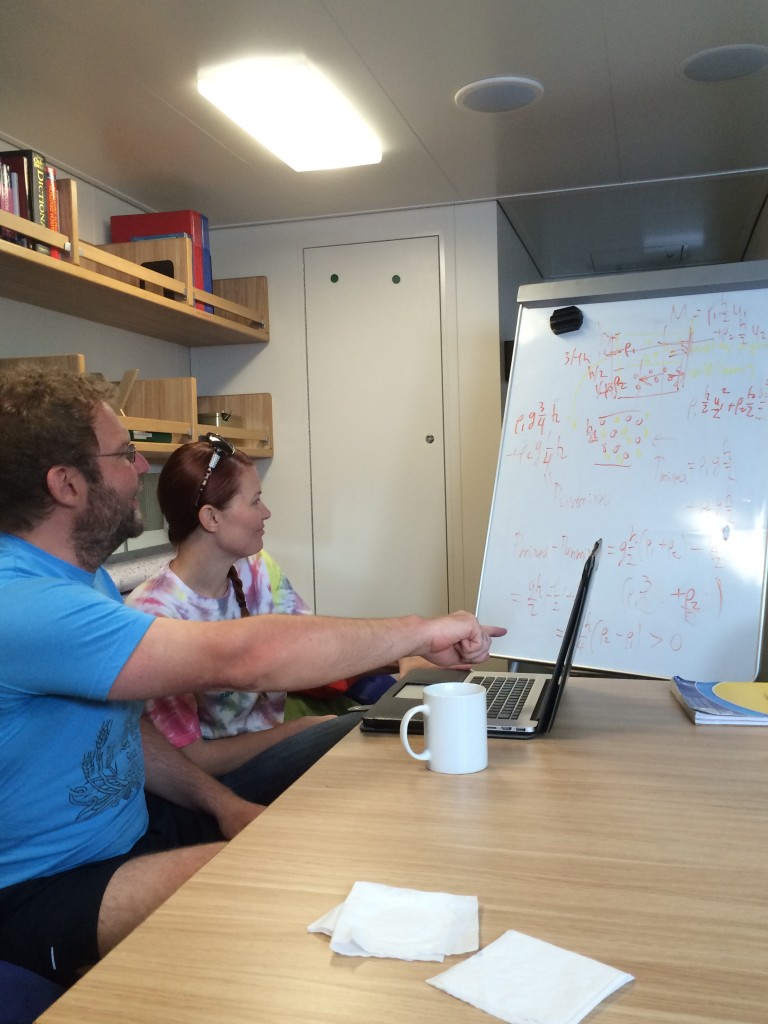
The energy needed to move the centers of gravity is present within the two layers, because moving fluid has velocity and therefore, kinetic energy. However, kinetic energy can only sometimes be converted into potential energy. “For example,” says Dr. Natarov. “When both layers are moving at the same velocity, kinetic energy of the flow cannot be converted into potential energy, because momentum is not conserved.” This is an example of the absence of shear.
When we calculate mixing, the energy and momentum conservation laws must be satisfied. Shear, which by definition requires layers of different velocities, is necessary for converting kinetic energy into potential energy without violating the conservation of momentum. Ultimately, using the relative densities of adjacent layers of fluid, we can calculate how much energy is needed for mixing the layers.
The bottom line
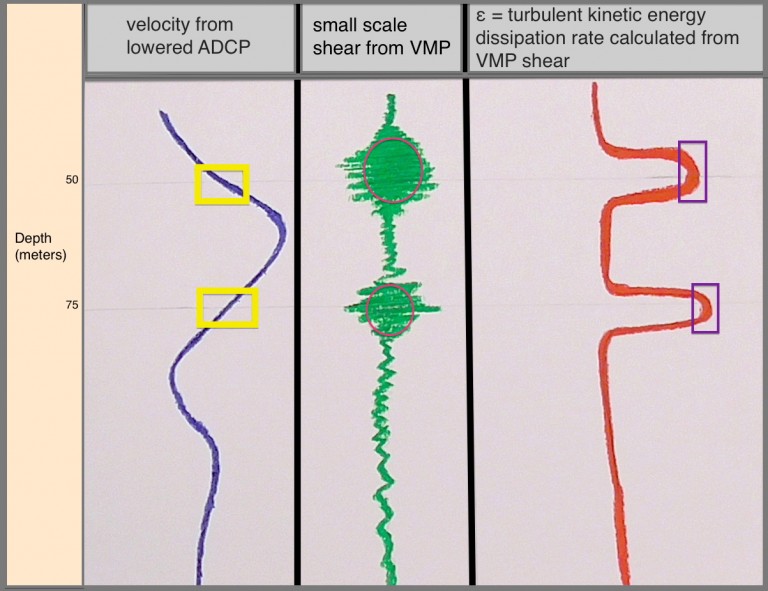
Large-scale shear produces flow instability, leading to turbulence, which causes mixing and therefore dissipation of energy. This is what the science team is after on this cruise. The Vertical Microstructure Profiler (VMP) measures the dissipation rate of the kinetic energy, i.e. the energy lost to the process, and provides the science team with a profile of shear on a much smaller scale than what they could measure on their own. Rapid velocity changes on a large-scale correlate with small-scale shear action and rapid energy dissipation at particular depths. The graph to the left helps visualize the difference between the small- and large-scale shear.
In the diagram to the right, the blue line represents current velocity as measured by the lowered ADCP, another instrument used on this cruise. The boxes on the blue line show where velocity changes rapidly, i.e., regions of large-scale shear. These regions correlate with areas of high shear activity as measured on a centimeter scale by the VMP, represented in green. The science team can then calculate the rate of turbulent kinetic energy dissipation (ε). The peaks diagramed in red, are considered to be a gauge of mixing (ε). Together, these provide a visual representation of the measurements being taken from Falkor and how shear relates to each instrument onboard.